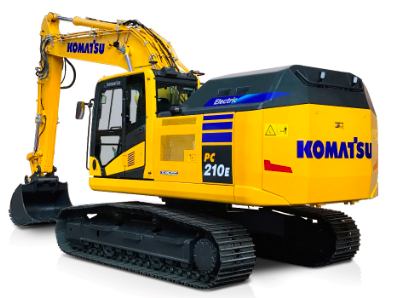
Battery-operated vehicles (BOVs), commonly known as electric vehicles (EVs), have gained immense popularity as a promising solution to combat climate change and reduce pollution. They offer the prospect of cleaner, greener transportation with zero tailpipe emissions. However, a critical question looms: Are BOVs truly the perfect solution to replace the internal combustion engine (ICE), or do they have hidden environmental costs associated with battery manufacturing and disposal? In this blog, we will explore both sides of the debate surrounding the environmental impact of BOVs.
The Promise of Electric Vehicles
Electric vehicles have several undeniable advantages:
Zero Tailpipe Emissions
BOVs produce no tailpipe emissions, contributing to cleaner air quality and reduced urban pollution. The key to their zero tailpipe emissions lies in how they generate and use that energy. Here's how it works:
Electric Power Source: EVs use electricity as their primary power source instead of internal combustion engines (ICEs) that rely on fossil fuels like gasoline or diesel. Electricity can be generated from a variety of sources, including renewable and non-renewable energy.
Clean Energy Sources: When electric vehicles are charged using electricity generated from clean and renewable energy sources such as wind, solar, hydroelectric, or nuclear power, the process of generating that electricity produces little to no greenhouse gas emissions. These sources are considered "clean" because they do not release carbon dioxide (CO2) or other pollutants into the atmosphere.
Zero Tailpipe Emissions: Unlike ICE vehicles, which burn fossil fuels and emit tailpipe pollutants such as CO2, nitrogen oxides (NOx), carbon monoxide (CO), and particulate matter (PM), electric vehicles do not have a tailpipe. They run entirely on electricity, and the emissions associated with the electricity generation happen at power plants, which are typically located away from urban areas.
Reduced Energy Waste: Electric motors are highly efficient at converting electrical energy into mechanical energy. They waste less energy in the form of heat compared to internal combustion engines, which are less efficient and release a significant portion of the energy as heat from the tailpipe.
Lower Energy Conversion Losses: The energy conversion process in electric vehicles involves fewer energy losses compared to ICE vehicles. Electric vehicles can use regenerative braking systems, which capture and convert some of the energy typically lost as heat during braking back into electrical energy, improving overall efficiency.
It's important to note that the "zero tailpipe emissions" claim for electric vehicles hinges on the source of the electricity used for charging. If an electric vehicle is charged primarily with electricity generated from fossil fuels, such as coal or natural gas, there will still be emissions associated with its operation, albeit significantly fewer than an equivalent gasoline or diesel-powered vehicle.
Lower Operating Costs Operating an electric vehicle (EV) can be significantly cheaper than operating an internal combustion engine (ICE) powered motor vehicle in several key ways. However, the cost savings associated with EVs can vary depending on factors such as the cost of electricity, fuel prices, driving habits, and the initial purchase price of the vehicle. Here are some reasons why operating an EV can be cheaper:
Lower Fuel Costs: Electricity is typically cheaper than gasoline or diesel fuel on a per-mile basis. The cost of charging an EV is generally less than the cost of filling up a tank of gas, especially when you consider that EVs are more energy-efficient than ICE vehicles.
Reduced Maintenance Costs: EVs have fewer moving parts than ICE vehicles, which means they require less maintenance. There are no oil changes, transmission fluid replacements, or exhaust system repairs. This can result in significant long-term savings.
Regenerative Braking: Many EVs are equipped with regenerative braking systems that capture and convert kinetic energy back into electricity when braking. This not only increases energy efficiency but also reduces wear and tear on brake pads, further lowering maintenance costs.
Lower Energy Loss: Electric motors are more efficient at converting energy into motion than internal combustion engines. This means that more of the energy from the battery is used to propel the vehicle, resulting in fewer energy losses and increased efficiency.
Incentives and Tax Credits: Many governments and regions offer incentives, rebates, and tax credits to encourage the adoption of electric vehicles. These financial incentives can significantly reduce the upfront cost of purchasing an EV.
Reduced Emissions Testing: Some areas require emissions testing for ICE vehicles, which can incur additional costs. EVs, with zero tailpipe emissions, are exempt from these requirements.
Long-Term Savings: Over the lifespan of an EV, the cumulative savings from lower fuel and maintenance costs can offset the typically higher upfront purchase price compared to ICE vehicles. As EV technology advances and becomes more widespread, prices are also expected to continue to drop.
It's important to note that the cost savings associated with EVs can vary depending on individual circumstances. Factors such as the local cost of electricity, driving habits, and the availability of charging infrastructure can impact the overall cost-effectiveness of owning an EV.
Additionally, while the operating costs of EVs are generally lower, the upfront purchase price of some EV models may be higher than that of equivalent ICE vehicles. However, as EV technology advances and economies of scale are realized, the price gap is expected to narrow, making EVs an increasingly attractive and cost-effective option for consumers.
Energy Efficiency electric vehicles (EVs) typically offer greater energy efficiency compared to internal combustion engine (ICE) vehicles. Several factors contribute to the higher energy efficiency of EVs:
Electric Motors vs. Internal Combustion Engines: Electric motors are inherently more energy-efficient than internal combustion engines. They can convert a higher percentage of the electrical energy from the grid into vehicle motion. In contrast, ICE vehicles waste a significant portion of their energy as heat, especially during combustion and through friction in the engine.
Regenerative Braking: Many EVs are equipped with regenerative braking systems, which capture and convert kinetic energy back into electricity when the vehicle slows down or brakes. This technology increases energy efficiency by recapturing energy that would be lost as heat in traditional braking systems.
Simplified Drivetrain: EVs have simpler drivetrains with fewer moving parts compared to ICE vehicles. There is no need for complex transmissions, clutches, or exhaust systems. Fewer components mean fewer energy losses due to friction and heat, resulting in improved efficiency.
Instant Torque: Electric motors deliver full torque instantly, even at low speeds. This provides efficient and immediate acceleration, reducing the need for excess energy consumption during acceleration phases.
Energy Use While Idling: EVs consume very little energy when idling. Unlike ICE vehicles, which need to keep the engine running at idle, EVs can shut down completely when not in motion, conserving energy.
Enhanced Aerodynamics: Some EVs are designed with improved aerodynamics to reduce air resistance at higher speeds. This contributes to better energy efficiency on highways.
Variable Power Delivery: EVs can vary the power delivered to the wheels more precisely than ICE vehicles. This means they can adapt power output to meet the driving conditions and maximize efficiency.
Energy Recovery: In addition to regenerative braking, EVs can recover energy during coasting or descending hills. This energy recovery feature further improves overall energy efficiency.
The Environmental Challenges of Batteries
However, the environmental challenges associated with batteries cannot be ignored:
Manufacturing Emissions:
The mining, extraction, and processing of raw materials like lithium, cobalt, and nickel can have significant environmental impacts, including habitat disruption, water contamination, and energy consumption. These materials are essential components of lithium-ion batteries, which power many electric vehicles (EVs) and portable electronic devices. Here are some of the key environmental concerns associated with their production:
Habitat Disruption: The mining of lithium, cobalt, and nickel often requires the excavation of large areas of land, which can lead to habitat disruption and destruction. This can have adverse effects on local ecosystems and biodiversity, especially in regions with sensitive or unique habitats.
Water Usage and Contamination: Mining and processing these materials can be water-intensive processes. The extraction of minerals can require significant amounts of water, which may lead to competition with local communities and ecosystems for this vital resource. Additionally, the chemicals and processes used in mining and refining can lead to water pollution and contamination if not properly managed.
Energy Consumption: The mining, extraction, and processing of these raw materials are energy-intensive processes. The energy required for drilling, blasting, crushing, and refining can contribute to greenhouse gas emissions if the energy is sourced from fossil fuels. This carbon footprint can partially offset the emissions reductions achieved by using EVs powered by lithium-ion batteries.
Chemical Use: Chemicals are often used in the extraction and processing of these materials. The improper handling and disposal of these chemicals can result in soil and water contamination, posing risks to local ecosystems and communities.
Human Rights Concerns: Cobalt, in particular, has been associated with human rights issues, including child labor and unsafe working conditions, in some mining regions. Efforts are being made to address these concerns and ensure responsible sourcing of cobalt.
Waste Management: The mining and refining processes generate waste materials, including tailings and slag. Managing and disposing of these wastes can be environmentally challenging, as they may contain toxic substances or heavy metals that can leach into the environment if not properly contained.
Efforts are underway to address these environmental challenges associated with the production of raw materials for batteries. Sustainable mining practices, recycling of battery materials, and responsible sourcing of minerals are becoming increasingly important. Additionally, advancements in battery technology, such as the development of alternative chemistries with less reliance on scarce or environmentally impactful materials, are being explored to reduce the environmental footprint of battery production.
As the demand for lithium, cobalt, and nickel continues to grow with the increasing adoption of EVs and renewable energy storage systems, it is crucial to prioritize environmentally responsible and sustainable practices throughout the entire supply chain to minimize the environmental impact of these materials.
Energy Intensive:
The manufacturing of lithium-ion batteries is energy-intensive. The production of lithium-ion batteries involves multiple energy-intensive processes, from the extraction and processing of raw materials to the assembly of battery cells and packs. Here are some key reasons why lithium-ion battery manufacturing is energy-intensive:
Raw Material Processing: The extraction and processing of raw materials like lithium, cobalt, and nickel require significant energy inputs. This includes mining, refining, and processing these materials to create the active components of the battery, such as cathodes, anodes, and electrolytes.
Electrode Production: Manufacturing the electrodes (the positive and negative terminals) of lithium-ion batteries involves several energy-intensive steps, including mixing, coating, and drying electrode materials. These processes are essential for creating the electrodes' functional layers.
Cell Assembly: Assembling battery cells involves the precise placement of electrode materials, separators, and electrolytes in a controlled environment. This assembly process requires energy for equipment operation, temperature control, and maintaining cleanroom conditions.
Formation and Testing: After assembly, lithium-ion cells undergo formation, where they are charged and discharged multiple times to stabilize their performance. This process consumes energy and time.
Quality Control: Ensuring the quality and safety of lithium-ion batteries involves rigorous testing procedures, including electrical and thermal testing, which require energy inputs.
Packaging and Transportation: The packaging and transportation of battery cells and packs also consume energy, especially in cases where the materials need to be transported long distances.
Recycling: Recycling of lithium-ion batteries is energy-intensive, as it involves the disassembly of battery packs, the separation of components, and the recovery of valuable materials.
It's worth noting that the energy intensity of lithium-ion battery manufacturing can vary depending on the scale of production, the efficiency of manufacturing processes, and the source of energy used. Facilities that rely on electricity generated from fossil fuels will have a higher carbon footprint compared to those powered by clean and renewable energy sources.
Efforts are being made to improve the energy efficiency of battery manufacturing processes and reduce the environmental impact. This includes the development of more efficient production equipment, the optimization of material use, and a transition to renewable energy sources in manufacturing facilities. As the demand for lithium-ion batteries continues to grow with the rise of electric vehicles and renewable energy storage, sustainable and energy-efficient manufacturing practices will play a crucial role in minimizing the environmental footprint of battery production.
Limited Lifespan:
The batteries of electric vehicles (EVs) have a limited lifespan, and their performance tends to degrade over time. The lifespan and degradation rate of an EV battery depend on several factors, including the type of battery, usage patterns, temperature, and charging habits. Here's what you need to know:
Battery Types: Lithium-Ion (Li-ion) Batteries: Most modern EVs use lithium-ion batteries, which are known for their high energy density and durability. Li-ion batteries typically have a longer lifespan compared to older battery technologies, such as nickel-metal hydride (NiMH) batteries.
Solid-State Batteries: Emerging solid-state battery technology has the potential to offer longer lifespans and improved performance compared to traditional Li-ion batteries, but it is still in the research and development phase for most consumer vehicles.
Factors Affecting Battery Lifespan:
Usage Patterns: The way an EV is driven and used can significantly impact battery lifespan. Frequent fast charging, deep discharging, and high-speed driving can contribute to faster battery degradation.
Temperature: Extreme temperatures, both hot and cold, can adversely affect battery performance and lifespan. It's essential to maintain the battery within an optimal temperature range.
Charging Habits: How an EV is charged can influence battery health. Avoiding frequent deep discharges and keeping the battery charge level within a moderate range (typically between 20% and 80%) can help prolong battery life.
Fast Charging: While fast charging is convenient, frequent use of high-power fast chargers can lead to accelerated battery degradation. Some EVs have thermal management systems to mitigate this.
Quality of Battery Management System (BMS): The effectiveness of the vehicle's BMS, which manages and balances the cells within the battery pack, can impact battery health. High-quality BMS systems can help optimize charging and discharging patterns to extend battery life.
Battery Degradation:
Over time, all lithium-ion batteries will experience some degree of degradation. This means that they will hold less charge and have reduced driving range compared to when they were new. Battery degradation is typically measured in terms of capacity loss, expressed as a percentage of the original capacity. For example, a battery with 80% capacity remaining has experienced 20% degradation.
Battery Warranty:
Many EV manufacturers provide warranties for their batteries to assure buyers of their performance and lifespan. These warranties often cover a specific number of years or miles and guarantee a certain level of capacity retention. For example, a common warranty might cover the battery for eight years or 100,000 miles, with a requirement that it retains at least 70-80% of its original capacity during that time.
In summary, while EV batteries do have a limited lifespan and experience degradation, they are designed to remain functional for a substantial period, often exceeding the typical ownership period of a vehicle. Proper care and charging habits can help maximize battery life, and warranty coverage provides additional peace of mind for EV owners. Additionally, ongoing research and development are focused on improving battery technology to extend both lifespan and performance.
End-of-Life Disposal:
Proper disposal or recycling of lithium-ion batteries is crucial to prevent toxic materials from entering the environment. However, without appropriate recycling infrastructure, there is a risk of environmental contamination.
The reality of lithium-ion battery disposal varies depending on region, regulations, and available recycling infrastructure. While recycling lithium-ion batteries is essential to mitigate environmental impacts, the reality is mixed:
Responsible Recycling:
Developed Countries: In many developed countries, there are established recycling processes for lithium-ion batteries. Battery recycling facilities are equipped to recover valuable materials like lithium, cobalt, nickel, and copper from old batteries. These materials can be repurposed for use in new batteries or other industries.
Battery Manufacturers: Some battery manufacturers have implemented take-back programs, encouraging consumers to return old batteries for recycling. These programs are designed to ensure that end-of-life batteries are handled responsibly.
Recycling Rate: The recycling rate for lithium-ion batteries in some developed regions can be relatively high, with a significant percentage of batteries being collected and recycled.
Challenges and Concerns:
Global Disparities: The reality is that recycling practices and infrastructure vary significantly around the world. In some regions, especially developing countries, there may be limited recycling facilities and resources dedicated to lithium-ion battery recycling.
Informal Recycling: In areas with inadequate recycling infrastructure, informal and often unsafe recycling methods may be employed. This can involve practices like open burning or the use of hazardous chemicals to recover valuable materials, leading to environmental pollution and health risks.
Collection Rates: Even in regions with recycling programs, the collection rate for lithium-ion batteries can be relatively low. Many old batteries end up in landfill waste, where they can pose environmental hazards.
Lack of Standards: The absence of consistent international recycling standards for lithium-ion batteries can complicate recycling efforts and hinder the responsible management of end-of-life batteries.
Environmental Concerns:
Improper disposal of lithium-ion batteries, whether through informal recycling or landfill disposal, can have negative environmental consequences. These may include:
Hazardous Materials Release: Batteries contain hazardous materials, including heavy metals like cadmium and lead. When batteries are not disposed of properly, these materials can leach into the environment, contaminating soil and water.
Air Pollution: Burning lithium-ion batteries can release toxic fumes and particulate matter into the air, contributing to air pollution and respiratory health risks.
Resource Depletion: Failing to recycle lithium-ion batteries means that valuable materials are not recovered and may need to be sourced through mining, increasing environmental impacts associated with resource extraction.
Efforts are being made to improve lithium-ion battery recycling globally, including the development of standardized recycling processes and the expansion of recycling infrastructure. However, there is still work to be done to ensure that the responsible recycling of lithium-ion batteries becomes the norm rather than the exception. Consumers can contribute to these efforts by participating in battery take-back programs and properly disposing of old batteries at designated collection points.
Balancing the Scales
It's essential to strike a balance and critically evaluate the environmental impact of BOVs:
1. Lifecycle Analysis: When assessing the environmental impact of BOVs, it's crucial to consider their entire lifecycle, including manufacturing, operation, and end-of-life. Studies have shown that even with battery manufacturing emissions considered, BOVs tend to have lower lifetime emissions compared to traditional ICE vehicles, especially when charged with clean, renewable energy.
2. Battery Advancements: Ongoing research and development efforts aim to create batteries that are more energy-efficient, have longer lifespans, and are easier to recycle. Advancements in battery technology may mitigate some of the environmental concerns associated with battery production and disposal.
3. Charging Infrastructure: The environmental impact of BOVs also depends on the source of electricity used for charging. A shift toward renewable energy sources can significantly reduce the emissions associated with EV operation.
4. Sustainable Materials: Efforts are underway to source materials more sustainably, reduce dependency on rare and environmentally impactful minerals, and improve recycling rates for batteries.
While battery-operated vehicles are not entirely without environmental impact, they remain a promising solution to replace the internal combustion engine in the fight against climate change and air pollution. The key lies in managing and reducing the carbon footprint associated with battery production and disposal, as well as transitioning to cleaner sources of electricity.
BOVs are not the "perfect" solution, but they represent a crucial step toward a greener and more sustainable transportation future. As technology continues to advance and environmental regulations tighten, the overall environmental impact of BOVs is expected to decrease. However, it's important to view them as one component of a broader strategy to reduce emissions, alongside improvements in public transportation, cycling infrastructure, and urban planning.
#heavyequipment#construction#excavator#heavymachinery#constructionequipment#heavyequipmentlife#caterpillar#earthmoving#mining#excavation#komatsu#equipment#heavyequipmentnation#cat#digger#excavators#demolition#heavyequipmentoperator#machinery#constructionlife#engineering#truck#concrete#bulldozer#earthmovers#constructionmachinery#civilengineering
Comments